Research
Research Programme
Short and long term scientific perspective
The importance of clean energy generation and efficient energy consumption, as well as the requirement of lowering emissions does not need to be dwelled on. A prime example is fuel cell technology [1] , which enables efficient energy generation with only water as an “exhaust” when the fuel cell is run on hydrogen. In case of methane as fuel, CO and CO2 are produced as well, but the efficiency is higher as compared to methane combustion. Solid Oxide Fuel Cells (SOFCs) are based on more or less complex oxides [2], and the widespread use of SOFCs eventually relies on mastering problems that are related to oxide surface chemistry (CH4 reforming, oxygen activation and transport, hydrogen oxidation, interface effects, material stability, etc).
In addition to SOFCs the surfaces and interfaces of metal oxides are relevant in many other areas of chemistry, physics, and technology, including heterogeneous/environmental/photo-catalysis, sensors, microelectronic circuits, piezoelectric devices, corrosion protection coatings, self-cleaning/antibacterial surfaces etc. The surfaces of oxides are not just a simple truncation of the bulk [3]. The fact that half the crystal is missing often leads to significant restructuring of the atomic arrangement, the presence of surface states, segregation effects, etc. All these properties can significantly affect surface chemistry and other physico-chemical properties. This is particularly true for very thin (i.e., less than 10 nm) oxide films [4]. Modified oxides, i.e., oxides that are doped, multicomponent oxides, oxide/oxide interfaces and heterostructures, play an even more prominent role in technology, and their properties are even more complex.
Future advances in oxide-based technologies rely on a fundamental understanding of the surface chemistry and physics of the involved materials, and on identifying structure-function relationships [5 - 8]. Many different experimental and computational techniques are available and have been refined in recent years, with a pronounced push towards developing methods that are operational at higher pressures [9, 10]. The complexity of these materials, further amplified by the fact that they change under operating conditions, necessitates that a very wide array of methodologies and techniques are applied to make true progress with understanding one specific material and/or addressing one problem. This can only be achieved in a concerted effort of groups with specialized, yet complimentary expertise.
In its first funding period the SFB FOXSI has unified 10 research groups in surface/interface chemistry and physics and fuel cell technology, each with a research focus in oxide surfaces and interfaces, see Fig. 01-1. Obtaining a holistic view of these materials relies on the application of a broad range of experimental and theoretical methods (STM, AFM, TEM/SEM, PEEM/FIM, SXRD, XPS, AES, LEIS, PM-IRAS, FTIR, SFG, TPD, UV-Vis, PL, impedance measurements, DFT, etc.). This includes microscopic as well as spectroscopic methods, area-integrating and locally-resolved, both ex situ and in situ. For example, a detailed analysis of the atomic and electronic structure or ZrO2 ultrathin films required the application of STM, LEED, synchrotron XPS, TPD, and DFT. For studies of surface reactions the approach needs to be extended by, at least, PM-IRAS, MS and PEEM. Such a wide array of methodologies can clearly not be provided by one single research groups but requires large research units such as an SFB.
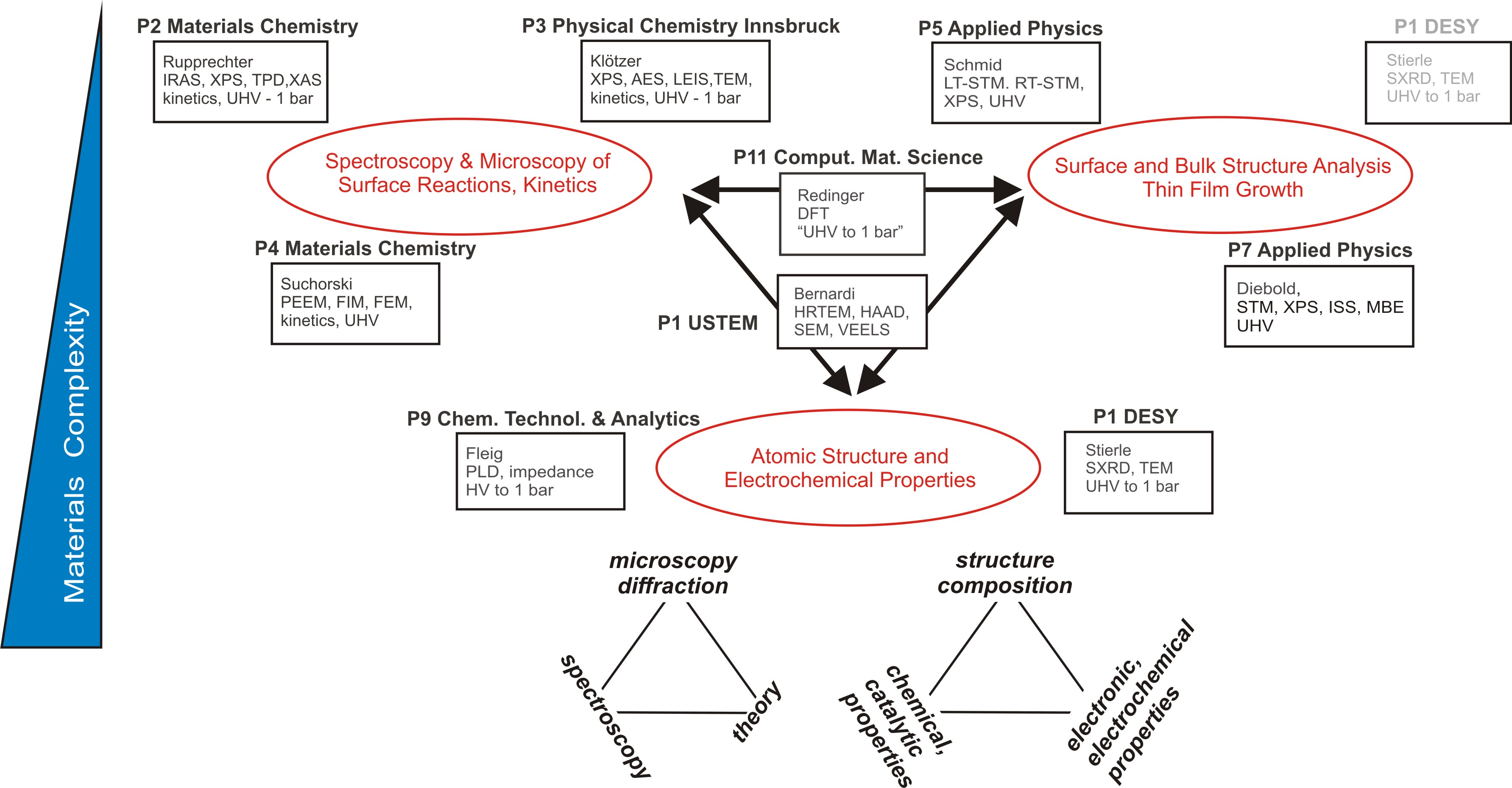
Figure 1-1 Project parts of the SFB FOXSI. Centers of interest are structural analysis (P5-P7), in situ monitoring of surface reactions (P2-P4), and advanced synthesis and electro-catalytic properties of complex oxides (P9-10) Theoretical modelling (P11) and electron microscopy characterization (P01) interconnect the three focal points and are relevant for all topics.
A key (and, as it turned out, very successful) component of the SFB FOXSI is its deliberate limitation to a small number of “Core Materials” and “Core Topics”. The materials that were chosen (ZrO2, pure modified by Y, CeO2, perovskites, and oxide-metal interfaces) are the functioning components in SOFCs [1, 2], and are important in many other areas as well. While the surface science of metal oxides has shown tremendous progress over the past two decades [11], little is understood about the surfaces our Core Materials, especially about ZrO2 and perovskites. The choice of Core Topics follows logically from the envisioned applications: (i) structure of functional oxide surfaces and interfaces; ii) gas-oxide interactions; iii) functional oxides under working conditions. This tight focus on a few selected systems and problems “pre-programmed” the close interactions between the participating groups. At the same time it provided enough “research space” so as to not stifle scientific creativity. The benefit of this thematic focus was, for example, pleasingly apparent during the Annual PhD workshops where the students dominated the intense discussion since all topics presented were rather closely related to their own PhD topic.
The concerted efforts of the SFB FOXSI during the first funding period have lead to the development of ultrathin ZrO2 model oxide films (with different defect density, and also modified by nanoparticles of Ni and other metals), and model perovskites, with a focus on fundamental insights into the atomic/electronic structure, chemical (catalytic) properties, and oxygen activation kinetics. In line with the original plan, studies of UHV grown model surfaces were paralleled by studies on corresponding technological materials. The theoretical component has been very important, as many of the experimental investigations were performed in close collaboration with theory. The tight interactions between the groups resulted in multiple student exchanges, collaborative beam times, and joint publications. Existing collaborations have been strengthened and new collaborations have been started; and all of them have become productive within a short period of time. As is described below, virtually each group has started working on systems that are new to them, and/or has established new methodologies. The investigations, many of them joint efforts, are in full swing and will continue, often in intensified form, in the second funding period. Again, the overall gaol of this 8-year project is to provide in-depth, physical insights relevant to functional oxide surfaces and interfaces, guided by the overarching objective to develop strategies for improving/optimizing the SOFC-relevant (catalytic) processes. We are well on our way.
Scientific development of the SFB
By setting the boundary conditions of Core Materials and Core Topics, the broad research work in SFB FOXSI has effortlessly channelled into three main thrusts: investigating the materials properties and function of (i) zirconia and related materials, (ii) perovskites, and (iii) development of novel methodologies, techniques, and research strategies. For (i) and (ii), the samples span a wide range of forms, from highly idealized and well-controlled single crystals toward more complex and “realistic” powders, with rapid feedback between these different approaches. In parallel, an assortment of experimental methods allows operating in pristine UHV conditions and in close-to ambient pressures, as well as in operando studies (see Fig. 01-2, below). The following gives a brief overview of this approach and some of the outcomes during the first funding period. The results are discussed in more detail in the reports of the individual project parts in section 4.
(ii) Zirconia:
• development of an ultrathin, epitaxial ZrO2 film as a model oxide with different, controlled levels of defects. The film forms through controlled oxidation/Zr segregation on top of Pt3Zr and Pd3Zr (111) single crystals
• studies of the adsorption of CO and H2O on these ultrathin ZrO2 model oxide films, in particular the effect of defects on water dissociation
• growth of various metal nanoparticles on these ultrathin ZrO2 films (including Ni, Fe, Pd, Au, Ag), with an emphasis of studies of the thermal stability of various metal nanoparticles
• ambient-pressure XPS studies of ultrathin model ZrO2 at BESSY and MaxLab
• initial stages of Zr oxidation by XPS and PEEM: three-component suboxides as an interlayer between the metal surface and a stoichiometric ZrO2 overlayer.
• domain-specific data for catalytic ignition in CO oxidation on individual Pd and Pt crystalline grains, and for Pd-powder supported by Al2O3 and ZrO2
• evaluation of corresponding technological materials (Ni, Cu, NiCu on ZrO2, YSZ, CeO2, CeO2-ZrO2 etc), in particular studies of the surface reactivities of YSZ, Y2O3, and ZrO2 powder samples towards H2, H2O, CO and CO2
• growth of thin film model systems of ZrO2 and Y2O3 with embedded Ni and Cu catalyst particles
• growth of hydroxylated and redox-active zirconia nanoclusters via ALD/CVD deposition of Zr(OtBu)4 on Pd(111) and Cu(111)
• formation of graphitic and nanotube like carbon structures on purely oxidic surfaces
• rates and selectivities of CH4 - and methanol reforming on Cu/zirconia catalyst systems
(ii) Perovskites:
• a full characterization of a ‘phase diagram’ for B-terminated reconstructions of SrTiO3 , and ab-initio thermodynamics for this system
• developed and characterized cleaved, SrO-terminated ruthenates as idealized model systems for A-site terminated perovskites, and found, and theoretically explained, its high chemical activity towards CO even under UHV conditions
• mapped the relevance of bulk and surface paths of oxygen reduction for various electrodes (LSM, LSC, Pt) and different temperatures and voltages
• quantified the effects of strain (LSC) and grain boundaries (LSM) on the oxygen reduction rate
• evaluated the importance of electronic conductivity in mixed conducting electrodes operated under reducing conditions and monitored the active zone and its voltage dependence
• determined rates and selectivities of CH4 and methanol reforming on Ni/perovskite and Cu/zirconia catalyst systems
• studied the surface reactivities of anode-relevant perovskites (LSF, STF) in the water gas shift reaction and their activation for methane reforming and methanation by metal doping
(iii) Methodologies
• developed synchrotron-based in-operando XPS/ impedance spectroscopy of perovskite model electrodes under electrochemical polarization in reducing or oxidizing atmosphere
• "local kinetics by imaging": local reaction kinetics on a m-scale from the PEEM images and on nm-scale from the FIM/FEM images.
• pioneered a method of using the field of a scanning tunnelling microscope to simultaneously initiate and observe motion of single O vacancies
• installed a Laser MBE system, integrated with a surface analysis chamber
• built a new apparatus with a molecular beam for TPD of oxide single crystals
• inclusion in DFT calculations of van der Waals interactions for interfaces (ZrO2 films) and adsorbates (CO and H2O)
• developed a UHV-compatible sputter source for Zr and zirconia/YSZ deposition
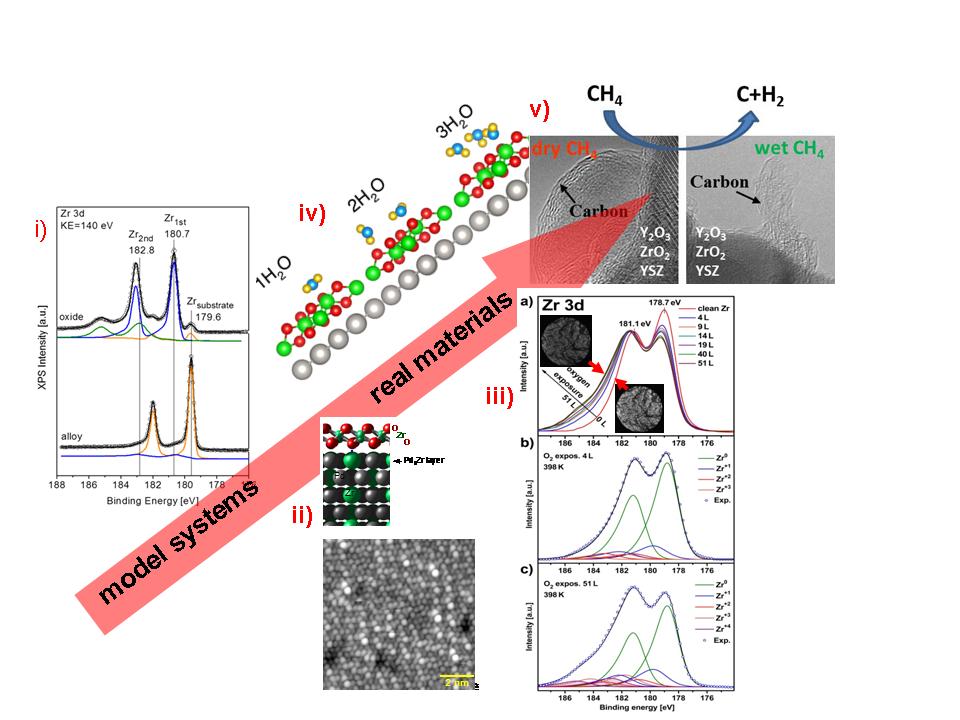
Figure 01-2. The SFB strategy at the example of structure and functionality studies on zirconia. (i) XPS Zr 3d spectrum of a clean Pt3Z alloy and an epitaxial, ultrathin ZrO2 trilayer film (P02), (ii) corresponding structures monitored by UHV-STM (P05) and modeled with DFT (P11) (iii) in situ XPS of the initial stages of polycrystalline Zr oxidation, the insets show corresponding PEEM images (P04) (iv) surface structure changes at increasing H2O coverage as modeled with DFT (P11) (v) carbon nanostructure growth on SOFC relevant oxides from methane-rich fuel (P03, P01)
Outcomes:
This project started on March 15, 2011, i.e., a little more than 3 years before this Report was written. During this time period, a total of 58 peer-reviewed papers were published; 27 of these papers were joint publications, involving at least two, sometimes more, Project Parts. Section 2.1.1. also lists ‘Papers in Preparation’, i.e, manuscripts that exist in a more or less publishable form. Of 45 papers that are ‘work in progress’, 30 are being written jointly. Clearly, this SFB has been productive right from its outset. The trend of publishing more and more papers together also reflects the collaborative spirit of this project: all participating groups have a desire to co-operate within the SFB that is driven by the scientific questions addressed.
List of References Cited
[1] S. C. Singhal, K. Kendall (Eds.), "High Temperature Solid Oxide Fuel Cells", Elsevier, 2003674 (2010).),
[2] A.J. Jacobson, Chem. Mater 22 (2010) 660
[3] U. Diebold, S.C. Li, and M. Schmid. Annu. Rev. Phys. Chem. 61 (2010) 129.
[4] G. Pacchioni and H-J. Freund, Chem. Rev. 113 (2013) 4035.
[5] H.-J. Freund, Angew. Chem. Int. Ed. 36 (1997) 452.
[6] H.-J. Freund, Surf. Sci. 500 (2002) 271.
[7] C. T. Campbell, Surf. Sci. Rep. 27 (1997) 1.
[8] V.E. Henrich, P.A. Cox, “The Surface Science of Metal Oxides”, Cambridge, Cambridge University Press, 1994.
[9] G. Rupprechter, Adv. Catal., 51 (2007) 133
[10] H. Bluhm, M. Hävecker, A. Knop-Gericke, M. Kiskinova, R. Schlögl and M. Salmeron, MRS Bulletin, 32 (2007) 1022.
[11] C.T. Campbell and J. Sauer, Chem. Rev. 113 (2013) 3859